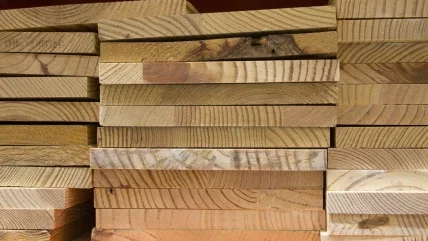
In the wood panels industry, most of us are working with resins in one way or the other. Whether we use it to glue solid wood panels together, to make fibreboard or coatings, there is usually some kind of resin involved. The less well-loved part among those who are working with resins is often the chemistry of it, where one must deal with complicated cross-linking reactions that are happening in the resin itself or between resin and wood polymers.
Fortunately, it is not always necessary to understand every last detail of these chemical reactions to use the product, but a solid theoretical background can help to optimise the application you are working with, for example, by adjusting your production line to new raw material.
If, for instance, a hypothetical company that has been joining softwood panels with a PRF resin system wants to extend the product portfolio to hardwoods, how do they know if they can use the same pressing temperature, time, and resin system? Of course, it would be possible to use mechanical tests, and let the test parameters like lap-shear or internal bond strength tell the story. But these tests consume much time, labour, and resources and are often inconclusive after all that work. Understanding the resin interaction with the wood might give a short cut to a better formulation.
To better understand the resin chemistry, we can use a technique called differential scanning calorimetry or DSC. This is a wellestablished thermoanalytical technique that is used to study polymers and resins. It can measure the glass transition, crystallisations, heat capacity, thermal stability, or curing kinetics of a polymer/resin. It can also be combined with other useful functionalities such as thermogravimetric analysis (TG), gas chromatography (GS-MS), or infrared (FTIR).
DSC analysis begins with a straightforward sample preparation, where a small sample of several milligrams must be placed in a crucible, typically using a pipette for liquids or a pair of tweezers for solids. The crucible can be made of aluminium, stainless steel, or ceramic depending on the temperature range that is under investigation.
One can use open crucibles that are in exchange with the environment or closed crucibles that can withstand high pressures. We will get back to this in a minute.
Once the sample is placed in the crucible, it is transferred to a furnace that contains two temperature sensors, one for the sample and one for an inert (empty) reference crucible. The furnace is then heated with a specified heating rate, typically between 5 – 30°C/min, and the temperatures of the sample and the reference are monitored. The difference between the two allows calculation of the heat flow that goes into the sample (endotherm) or comes out of it (exotherm).
The curing reaction of most resins is exothermic, but the analysis is complicated in water-borne resins, because the evaporation of water is endothermic, which causes an overlapping of both signals. Therefore, to study the curing reaction of water-borne resins, we need to use closed high-pressure crucibles. This way, the endothermic evaporation of water is prevented even at high temperatures, and it becomes possible to isolate the exothermic signal of the curing reaction.
The exothermic signal reveals the onset and peak temperature of the reaction, which can be used to estimate the best curing conditions in your process. The area under the exothermic signal in the graph gives the specific heat, which shows how much energy is released (or consumed) by the reaction.
Moreover, by testing the same sample at three different heating rates we can use the relationship between heating rate and peak temperature to learn more about kinetic parameters such as the reaction rate, reaction order, or activation energy.
So, the hypothetical company from earlier could simply run a few DSC scans comparing their resin in the presence of a softwood and a hardwood, calculate the curing kinetics, and would already have a good idea about whether to adjust their process parameters to the new raw material.